he impact of decoupling capacitors and a PCB trace length on signal integrity was discussed in [1], while the impact on radiated emissions was discussed in [2]. In this article, we evaluate the impact of the capacitors and trace length on conducted emissions.
Measurements were taken on both the battery and ground lines, in the frequency range 150 kHz – 108 MHz. In the frequency range 150 kHz – 30 MHz measurements were taken with a bandwidth of 9 kHz while in the range 30 MHz – 108 MHz the bandwidth was set to 120 kHz. All measurements were taken with the average, peak and quasi-peak detectors.
30 – 42 MHz: Short trace outperformed the long trace, on both the battery and ground lines, using both detectors.
44 – 88 MHz: Long trace outperformed the short trace, on both the battery and ground lines, using both detectors.
90 – 108 MHz: Short trace outperformed the long trace, on both the battery and ground lines, using both detectors.
Ground Line: Capacitors eliminated the failures at 6.04 MHz and 26.17 MHz.
Ground Line: Capacitors eliminated the quasi-peak failures up to the frequency of 87 MHz, and the failures in the range 87 – 108 MHz were substantially reduced. The average detector failures were eliminated up to the frequency of 73 MHz, and the failures in the range 73 – 108 MHz were substantially reduced.
- Bogdan Adamczyk and Mathew Yerian-French, “Impact of a Decoupling Capacitor and Trace Length on Signal Integrity in a CMOS Inverter Circuit,” In Compliance Magazine, January 2024.
- Bogdan Adamczyk and Mathew Yerian-French, “Impact of a Decoupling Capacitor and Trace Length on Radiated Emissions in a CMOS Inverter Circuit,” In Compliance Magazine, March 2024.
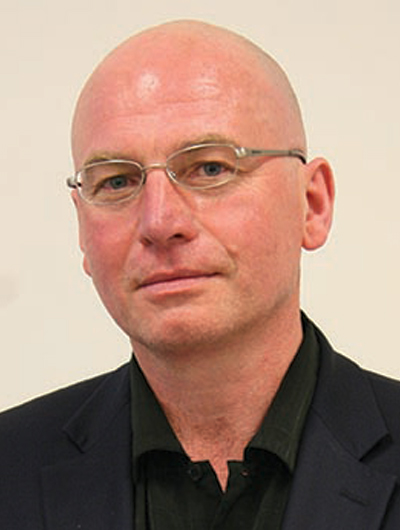
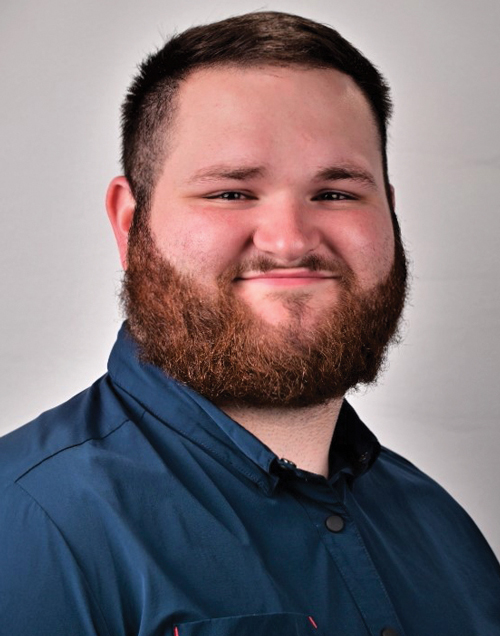